Quantum entanglement is a bizarre, counterintuitive phenomenon that explains how two subatomic particles can be intimately linked to each other even if separated by billions of light-years of space.
Despite their vast separation, a change induced in one will affect the other.
In 1964, physicist John Bell posited that such changes can be induced and occur instantaneously, even if the particles are very far apart. Bell’s Theorem is regarded as an important idea in modern physics, but it conflicts with other well-established principles of physics. For example, Albert Einstein had shown years before Bell proposed his theorem that information cannot travel faster than the speed of light. Perplexed, Einstein famously described this entanglement phenomenon as “spooky action at a distance.”
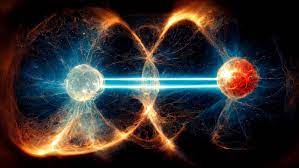
What is quantum entanglement?
Quantum entanglement is when a system is in a “superposition” of more than one state. But what do those words mean? The usual example would be a flipped coin. You flip a coin but don’t look at the result. You know it is either heads or tails. You just don’t know which it is. Superposition means that it is not just unknown to you, its state of heads or tails does not even exist until you look at it (make a measurement). If that bothers you, you are in good company. If it doesn’t bother you, then I haven’t explained it clearly enough.
You might have noticed that I explained superposition more than entanglement. The reason for that is you need superposition to understand entanglement. Entanglement is a special kind of superposition that involves two separated locations in space. The coin example is superposition of two results in one place. As a simple example of entanglement (superposition of two separate places), it could be a photon encountering a 50-50 splitter. After the splitter, the photon could be In path A, or it could be in path B. In this case, the superposition is between
- a photon in path A and no photon in path B.
and
- no photon in path A and a photon in path B.
As a normal human being, you think that it is in just one or the other, and it is just that you don’t know which. But in fact, it is in both, until you actually measure it. Again, that normal human being wants to say that if I measured it and found it in path A, it was in path A even if I hadn’t measured it. But making that assumption gets you into trouble. Assuming the particle has that definite characteristic before you actually measured it, leads to measured results that are just not possible.
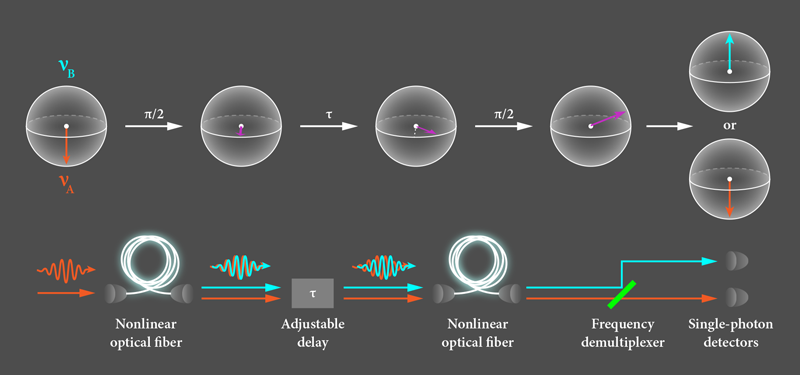
How does quantum entanglement work?
An example of quantum entanglement that I work with involves a light source that emits two photons at a time. Those two photons of a pair can be entangled so that the polarizations of the individual photons can be any orientation (i.e., random), but photons of a pair always have matching polarizations.
What is polarization? The polarization of light depends on the electric field of the light wave. As the light travels from point one point to another, its electric field will oscillate transversely to that propagation direction. It might oscillate in the vertical plane, in the horizontal plane or any direction in between.
Back to those entangled pairs. So, if I measure the polarization of photon A to see if it is polarized horizontal or vertical, I get an answer and find it to be, this time, vertical. Entanglement means that when I measure whether its twin is horizontal or vertical, I find that its polarization is vertical too. If I do that experiment many times, I will always find that the two photons’ polarizations match, even if I find that the result of which polarization they match to is random. (Think a pair of magical loaded dice.) So, a key point is that the measurement result will be random, but if I make the same measurement on the twin, I will get that same random result. (Again, as a normal human being, that should bother you.)
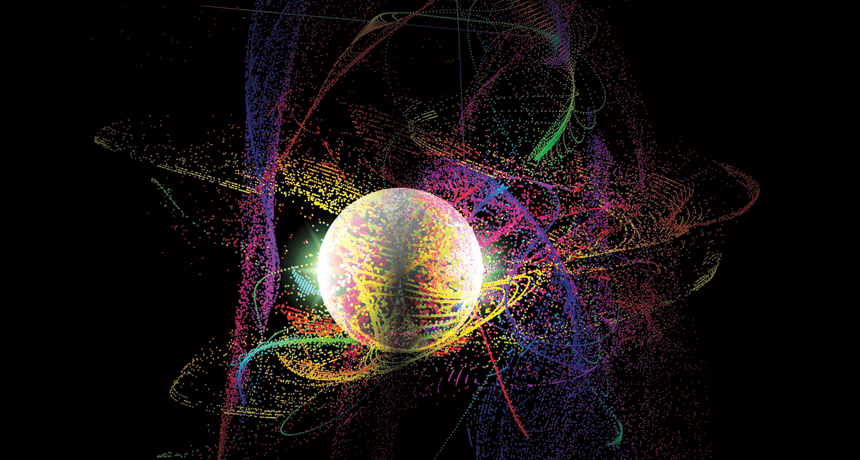
One of those studies was led by Krister Shalm, a physicist with the National Institute of Standards and Technology (NIST) in Boulder, Colorado. Shalm and his colleagues used special metal strips cooled to cryogenic temperatures, which makes them superconducting, meaning they have no electrical resistance. A photon hits the metal and turns it back into a normal electrical conductor for a split second, and scientists can see that happen. This technique allowed the researchers to see how if at all, their measurements of one photon affected the other photon in an entangled pair.